Inflammation is a natural whole-body response triggered by the immune system, which in turn can be generally divided into the innate and the adapted or acquired immune system. Both the innate and the adaptive immune system consist of different cell types, molecular signaling pathways, and cascades, all of which play specific roles during the immune response.
The innate immune system is a rudimentary first line of defense, responsible for initiating the inflammatory response.
The adaptive immune system is more highly evolved. designed to learn and create memory as the organism is exposed to antigens throughout its life.1
The immune system performs multiple essential tasks. It is a defense mechanism to recognize, fight, and eliminate pathogens and foreign material from the body. The immune response is crucial for the initiation of wound and fracture healing, tissue repair- and reconstitution, and the re-establishment of tissue homeostasis after injury.2-4
In general, the inflammatory response can be divided into three phases:
• acute,
• subacute,
• and chronic inflammation.
These phases differ in their cellular content and inflammatory signaling pathways as well as in their duration. Acute inflammation is triggered immediately by a stimulus and usually lasts only a few days.
Chronic inflammation can last months or even years when the acute inflammatory response is not resolved. The subacute phase describes a transitional period from acute to chronic inflammation and typically lasts several weeks.5,6
The acute inflammatory response: A quick, strong, and efficient process to re-establish tissue homeostasis after injury
Acute inflammation is triggered by the innate immune system. In case of tissue injury, it maintains tissue integrity and aids in the reconstitution of the tissue’s structural and physiological form and function. The acute inflammatory response is activated immediately after tissue injury and clinically characterized by five cardinal signs:
• heat (calor),
• redness (rubor),
• swelling (tumor),
• pain (dolor),
• and loss of function (functio laesa)1.
The cardinal signs of inflammation (calor, rubor, tumor, and dolor) were first described by the Roman writer Aulus Cornelius Celsus, and the fifth sign, (functio laesa), was added by the Roman physician Galen.7,8
One example is the injury of the skin caused by ascratch or stitch (Figure 1).
The tissue injury initiates the release of transmitters such as histamine from mast cells, which stimulate the dilation of blood vessels. This dilation reduces
blood flow velocity and increases the movement of blood in extremities, resulting in local heat and redness due to an enhanced number of red blood cells passing.1,9 Furthermore, the dilated blood vessels become more permeable, thus increasing the passage and accumulation of fluids in the surrounding tissues, which is manifested by swelling (edema). Release of specific mediators and edemainduced stretching of sensory nerves increases pain sensitivity in tissues containing nerve endings. The loss of function refers to either simple loss of mobility in a joint due to edema and pain or to the replacement of functional tissue with scar tissue.1,9,10
At this point it is important to mention that with tissue injury external (exogenous) pathogens such as bacteria, viruses, and other microorganisms can enter the site of injury. Intruding pathogens also release specific mediators and molecules to activate the immune system.11 Once pathogens are involved, it is called an infection.12
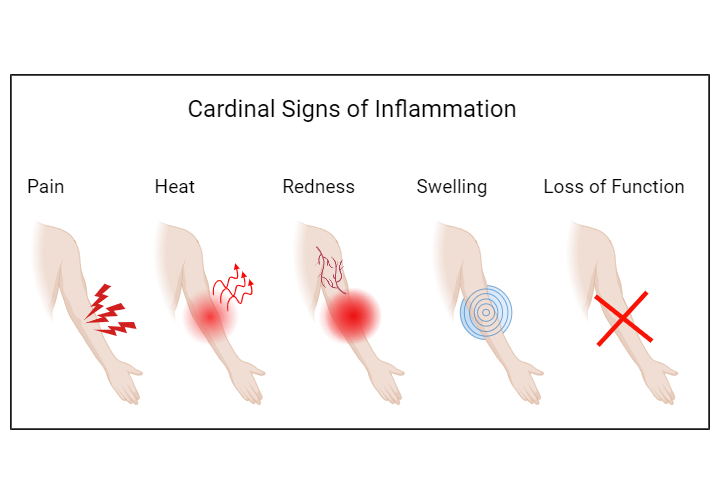
Fig. 1: The five cardinal signs of inflammation.
The acute inflammatory response is activated immediately after tissue injury. The above figure shows tissue injury in an example of a skin injury on the forearm. The clinical symptoms of the acute inflammatory response are pain (dolor), heat (calor), redness (rubor), swelling (tumor), and loss of function (functio laesa).
Figure created with BioRender.com, 2024.
Immune cells are the main actors during the acute inflammatory response following tissue injury.
With the initiation of the acute inflammatory response in the example of a penetrating skin injury, a cellular reaction cascade is triggered in the injured tissue, with immune cells being the main actors. During acute inflammation, various immune cells, cellular and molecular signaling pathways, and cascades aim to clear the site of tissue injury from damaged cells and/or pathogenic or foreign material and to induce tissue healing.13,14
At the site of tissue injury, local resident immune cells (so-called tissue macrophages) are activated. Macrophages are scavenger cells that play a crucial role in the inflammatory response. These cells have specific receptors on their surface which help them to identify external (exogenous) and internal (endogenous) material such as damaged tissue particles and cells (DAMPs), pathogens and their products (PAMPs), and other foreign material. When macrophages identify and recognize exogenous or endogenous particles by their specific surface receptors (PRRs), they become activated.3,13,15
Once activated, they start to engulf, digest, and degrade the particle by a process called phagocytosis, efficiently eliminating particles from the tissue. Macrophages also present parts of the digested particle on their surface to other immune cells, thus supporting and accelerating particle recognition.1,16 Furthermore, macrophage activation results in the release of pro-inflammatory mediators (cytokines and chemokines) into the tissue and blood, attracting more immune cells to the site of injury. This process is called chemotaxis.3,13
The dilation of blood vessels and their increased permeability facilitate the migration of additional immune cells into the injured tissue. Circulating neutrophils are attracted to the site of tissue injury, efficiently eliminating invading pathogens by the release of toxic material and by phagocytosis.13,17 Neutrophils are immune cells that survive only a couple of days, which later becomes important to the resolution of acute inflammation.13 Once damaged tissue, dead cells, pathogens, or foreign material are removed from the injured tissue, macrophages initiate the resolution of the inflammatory response.
Following the inflammatory phase, anti-inflammatory mediators and growth factors are released to suppress inflammation and initiate the proliferative phase.18 During the proliferative phase, several tissue repair events, including angiogenesis (formation of new blood vessels), granulation tissue (new tissue) formation, and re-epithelialization (re-establishment of surface layer) are initiated. New connective tissue is formed with neovascularization (new vessel formation), with fibroblasts being the key cells accountable for constructing granulation tissue to fill in the wound gap.13 Figure 2 shows the acute inflammatory response using the example of a enetrating skin injury .
Mast cells (purple cells) in subcutaneous tissue are activated and release transmitters such as histamine (purple dots), stimulating the dilation of blood vessels. Also, tissue-resident macrophages (green cells) become activated by particles from injured tissue and damaged cells (DAMPs) (pink dots). Activation of macrophages triggers the release of inflammatory cytokines (green dots) into the tissue. The macrophages start to engulf the damaged tissue particles.
Inflammatory cytokines and DAMPs attract additional immune cells to the site of tissue injury. Circulating neutrophils (light blue cells) migrate into injured tissue and start to remove tissue particles. Circulating monocytes (dark blue cells) also migrate into injured tissue and differentiate into macrophages. Neutrophils and macrophages engulf, digest, and remove damaged tissue particles, then release factors stimulating tissue healing (dark purple dots).
Macrophages release anti-inflammatory mediators (green dots), resulting in the resolution of the tissue inflammation. New blood vessels are formed (angiogenesis). Fibroblasts (brown cells) form new connective tissue.
Mast cells (purple cells) in subcutaneous tissue are activated and release transmitters such as histamine (purple dots), stimulating the dilation of blood vessels. Also, tissue-resident macrophages (green cells) become activated by particles from injured tissue and damaged cells (DAMPs) (pink dots). Activation of macrophages triggers the release of inflammatory cytokines (green dots) into the tissue. The macrophages start to engulf the damaged tissue particles.
Inflammatory cytokines and DAMPs attract additional immune cells to the site of tissue injury. Circulating neutrophils (light blue cells) migrate into injured tissue and start to remove tissue particles. Circulating monocytes (dark blue cells) also migrate into injured tissue and differentiate into macrophages. Neutrophils and macrophages engulf, digest, and remove damaged tissue particles, then release factors stimulating tissue healing (dark purple dots).
Macrophages release anti-inflammatory mediators (green dots), resulting in the resolution of the tissue inflammation. New blood vessels are formed (angiogenesis). Fibroblasts (brown cells) form new connective tissue.
Fig. 2: The acute inflammatory response. The acute inflammatory response is exemplified by a penetrating skin injury. Injury to cutaneous and subcutaneous tissue immediately triggers an acute inflammatory response.
This figure is adapted from “The Inflammatory Response,” created by Danielle Penk using BioRender.com, 2024. Retrieved from https://app.biorender.com/biorender-templates
From acute to chronic inflammation: The undesired immune response
The acute inflammatory response is a highly coordinated process, with different immune cells and various pro- and anti-inflammatory cascades tightly controlled in an equilibrium. Thus, the acute inflammatory response is a quick, strong, highly efficient process which usually lasts only a few days. In some cases, however, the acute inflammation does not resolve and progresses from subacute to chronic inflammation (Figure 3).5
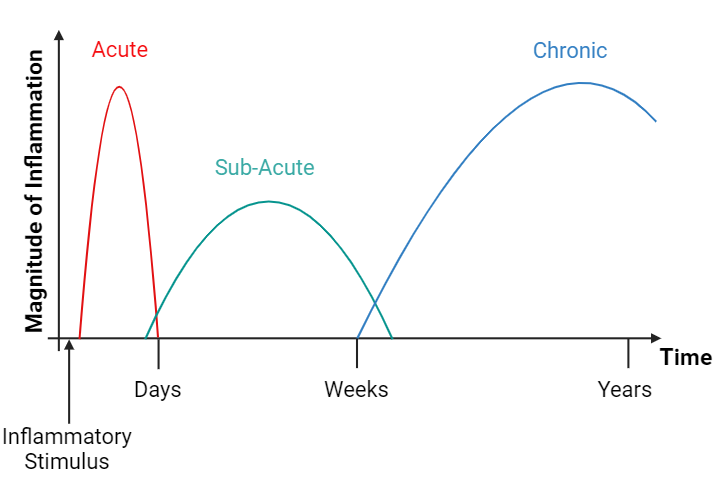
Fig. 3: Phases of inflammation.
Generally, inflammation can be divided into three phases: acute, sub-acute and chronic inflammation. After an inflammatory stimulus, the acute inflammatory response is immediately activated, a strong phase that usually lasts only a couple of days. If the acute inflammatory response does not resolve, it progresses from the sub-acute to the chronic stage, which can persist for a varying length of time.
Figure created with BioRender.com, 2024.
Chronic inflammation is characterized by continuous, unresolved, and uncontrolled activation of inflammatory cells and mediators, which differ from those of the acute inflammatory response. Chronic inflammation is referred as slow, long-term inflammation which can last for prolonged periods (months to years6). The causes of chronic inflammation can vary and are due to (but not limited to) failure of eliminating the agent causing the inflammation (infectious organisms), to constant low-level exposure of a foreign material, to an autoimmune disorder, to a defect in cells responsible for mediating the inflammatory response, to recurrent episodes of acute inflammation and to other inflammatory mediators.6
Most features of acute inflammation continue as the inflammation becomes chronic such as the dilation of blood vessels, increased blood flow, capillary (blood vessel) permeability, and migration of immune cells into affected tissue.6 However, the composition of immune cells changes drastically, and short-lived immune cells are replaced by cells from the adaptive immune system (macrophages, lymphocytes, and plasma cells). Thus, the hallmarks of chronic inflammation are infiltration of immune cells into affected tissue, which constantly release pro-inflammatory mediators, growth factors, and enzymes.6 Despite ongoing attempts at tissue repair, the constant and chronic activation and infiltration of immune cells lead to tissue damage (necrosis), granuloma (aggregate of immune cells) formation, and fibrosis (scar formation), eventually resulting in damaged or non-functional fibrotic tissue.3,6Irreversible tissue damage can further fuel inflammatory processes, weaken the immune system, and potentially predispose the body to other disease and infection.2
The body’s immune response to biomaterials and medical devices
The ability of a biomaterial to perform its intended function in the body depends on many factors, but the ultimate determinant of its success or failure is the host response.19 The host reaction begins immediately after implantation and consists of the reaction to the surgically induced tissue injury and to the material itself. Usually, the response to surgery-related tissue injury resolves quickly as part of the wound healing process.19 The reaction to the material lasts as long as the material is present in the body and depends on several factors related to either the material or the host.19 Inflammation, healing, and foreign body reaction (FBR) are the earliest host responses following implantation.20,21
The process of wound healing after total joint arthroplasty surgery
Total joint arthroplasty (TJA) is a successful technique to replace and preserve the form and function of major joints such as hip, knee, and shoulder. The majority of today’s orthopedic implants have good biocompatibility and osseointegration potential and a controlled implantation-induced inflammatory response. Wound healing is a normal biological process that takes place in four precisely programmed phases:
Hemostasis,
inflammation,
proliferation,
and remodeling.22
For proper wound healing, all four phases must occur in the correct order and time frame.22 Here, we focus particularly on the inflammatory phase during wound healing after TJA.
After TJA, surgery-related tissue injury triggers an inflammatory response and a sequence of events in the surrounding tissue with the aim of wound healing and proper reconstitution of tissue at the implant site.23Table 1 lists the sequence of the host events following tissue injury in periprosthetic soft- and bone tissue. It is important to mention that the inflammatory response and wound healing process after biomaterial implantation is dependent on the tissue- and site of implantation. Therefore, the wound healing process and FBR of subcutaneously implanted materials can differ from those that take place in the periprosthetic soft and bone tissue after TJA.20,23,24 In this review we are focusing on the latter. The process of periprosthetic soft and bone tissue are demonstrated in figure 4 and 5.
After the implantation of a periprosthetic device, blood-material interactions occur with protein adsorption on the biomaterial surface and the development of a thrombus-blood clot at the tissue-material interface. An injury to the vascularized tissue during the implantation procedure immediately activates the innate immune system and initiates blood coagulation. Complex reaction cascades of blood coagulation and the innate immune response result in the formation of a thrombus on the implant surface. This thrombus is considered a provisional matrix, providing structural, biochemical, and cellular components, which are important for the processes of wound healing and foreign body reaction. The formation of a provisional matrix is usually completed within a week, followed by the phases of acute and chronic inflammation in a sequential fashion.20,23,25
The acute inflammatory phase is characterized by the infiltration of short-living immune cells and mast cell degranulation. These immune cells release pro-inflammatory mediators and vasoactive substances, attracting and recruiting other immune cells, particularly macrophages to the site of injury. The phase is usually completed within a week.20
The phase of chronic inflammation follows the acute inflammatory phase. It is important to mention that this phase is specific for the tissue wound healing process and differs from the clinical definition, describing a slow, long-term inflammation which can last up to several years. Chronic inflammation during periprosthetic wound healing typically lasts two to three weeks. This phase is characterized by the infiltration of monocytes, which differentiate in the tissue into macrophages, and of other white blood cells (lymphocytes). The phase of chronic inflammation is followed by the formation of granulation tissue. Granulation tissue is a specific kind of tissue which is the hallmark of healing. It derives its name from the pink, soft, granular appearance on the surface of healing wounds. This tissue is characterized by formation of new small blood vessels and by the presence of macrophages and fibroblasts, which produce new connective tissue. The formation of granulation tissue eventually results in healing of the joint capsule.23
Importantly, the persistence of an inflammatory response beyond three weeks may indicate an infection, the onset of an abnormal reaction to the implant, or a combination.
This phase is unusual during the wound healing process in the joint. The formation of so-called foreign body giant cells (FBGCs) will be addressed later in this review. It is important to mention that, during the process of periprosthetic wound healing, this cellular reaction only occurs in presence of orthopedic cement. The formation of FBGCs usually starts later in the presence of wear particles from the implant. Nanoparticulate wear debris may be present earlier in periprosthetic tissue after joint implantation but is difficult to quantify due to the limited availability of clinical samples.
Phases of periprosthetic bone reaction
Generally, peri-implant bone healing is analogous to intramembranous bone healing after fracture. It is composed of two phases: an early phase (consisting of phases 1-4) and a late phase (consisting of phase 5). The phases of periprosthetic bone healing after joint implantation have mostly been investigated in experimental but not in clinical studies.
Similar to the periprosthetic tissue, the phase of chronic inflammation is characterized by the presence of monocytes, which differentiate into macrophages. Additionally, various signaling molecules such as pro-inflammatory mediators, growth factors, and angiogenic factors are released into the peri-implant space. Thisresults in the recruitment, migration, and differentiation of mesenchymal stem cells (MSCs), which are important for woven (primary) bone formation.26
MSCs differentiate either into bone-forming cells (osteoblasts), forming immature primary (woven) bone or into fibroblasts, forming fibrous membrane at the implant surface.27 Two types of bone formation (osteogenesis) occur: a) contact osteogenesis directly on the surface of the implant, and b) distance osteogenesis from the tissues surrounding the implant.28
After formation of woven bone, bone remodeling occurs through specific bone cells (osteoclasts and osteoblasts), gradually transforming the provisional woven bone into the lamellar bone. This dynamic process occurs for one year or longer and is necessary for successful long-term fixation.27
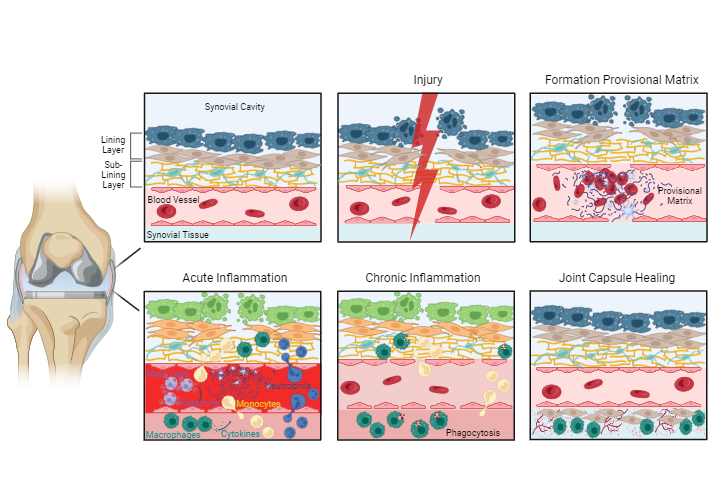
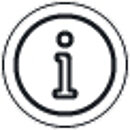
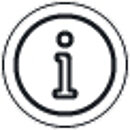
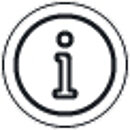
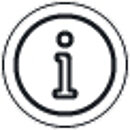
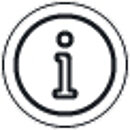
During surgery, the synovial membrane and the synovial tissue with blood vessels get disrupted, resulting in tissue injury.
Following injury, the immune system and the coagulation system get immediately activated and a blood thrombus (blue scaffold) with erythrocytes (red blood cells) and activated platelets (light blue cells) is formed, which provides a provisional matrix.
Mast cells (purple cells) are activated, releasing histamine (purple dots) into tissue, stimulating dilation of blood vessels. Tissue-resident macrophages (dark green cells) become activated, releasing inflammatory cytokines (green dots) into tissue. Neutrophils (blue cells) and monocytes (yellow cells) are attracted and migrate into injured tissue. Lining macrophage-like synoviocytes (light green cells) and fibroblast-like synoviocytes (brown cells) become activated in synovial membrane. Macrophages and neutrophils in injured tissue start to engulf and digest damaged tissue particles.
Monocytes migrate into injured tissue, differentiating into macrophages. Macrophages digest and remove damaged tissue particles and induce tissue healing.
Granulation tissue (pink tissue with pink dots) is formed by fibroblasts (brown cells). New blood vessels are formed (angiogenesis).
During surgery, the synovial membrane and the synovial tissue with blood vessels get disrupted, resulting in tissue injury.
Following injury, the immune system and the coagulation system get immediately activated and a blood thrombus (blue scaffold) with erythrocytes (red blood cells) and activated platelets (light blue cells) is formed, which provides a provisional matrix.
Mast cells (purple cells) are activated, releasing histamine (purple dots) into tissue, stimulating dilation of blood vessels. Tissue-resident macrophages (dark green cells) become activated, releasing inflammatory cytokines (green dots) into tissue. Neutrophils (blue cells) and monocytes (yellow cells) are attracted and migrate into injured tissue. Lining macrophage-like synoviocytes (light green cells) and fibroblast-like synoviocytes (brown cells) become activated in synovial membrane. Macrophages and neutrophils in injured tissue start to engulf and digest damaged tissue particles.
Monocytes migrate into injured tissue, differentiating into macrophages. Macrophages digest and remove damaged tissue particles and induce tissue healing.
Granulation tissue (pink tissue with pink dots) is formed by fibroblasts (brown cells). New blood vessels are formed (angiogenesis).
Fig. 4: Periprosthetic soft tissue reaction after TJA.
After total or partial joint arthroplasty, surgery-related tissue injury triggers an inflammatory response and thus a sequence of events in the surrounding tissue with the aim of wound healing and proper reconstitution of tissue at the implant site. Periprosthetic soft tissue healing is exemplified in the synovial membrane and the synovial soft tissue of a knee joint. The synovial membrane comprises a lining layer with lining macrophage-like synoviocytes (dark blue cells) and lining fibroblast-like synoviocytes (brown cells). The sub-lining layer comprises extracellular matrix (yellow scaffold) and telopode-bearing telocytes (mint green cells). The inflammatory status in the synovial membrane before partial or total joint arthroplasty surgery is presented in an oversimplified way. Patients receiving partial or total joint arthroplasty due to osteoarthritis usually exhibit varying degrees of chronic inflammation in the synovium before surgery.
Figure created with BioRender.com, 2024.
Adverse Local Tissue Reactions (ALTRs)
Although orthopedic implants have good biocompatibility and osseointegration potential, adverse local tissue reactions (ALTRs) can occur. With imaging techniques, ALTRs appear as thickened pseudocapsules and extraarticular fluid extensions.29,30 ALTRs comprise a range of histological patterns, ranging from purely macrophagic to mixed lymphocytic and macrophagic with or without features associated with hypersensitivity, and predominant sarcoid-like granulomas.31
The main symptoms of ALTR are pain and swelling. ALTR can result in extensive destruction of joint tissue, challenging the prognosis for further clinical solutions.32-34 ALTRs are described as soft or solid masses, in which the loss of the synovial surface with or without fibrin deposition, is accompanied by sub superficial necrosis, mononuclear cell infiltration and variable number of immune cells and giant multinucleated cells (FBGCs), in a thickened synovial membrane composed of dense connective tissue.34-37 ALTRs are associated with aseptic loosening and implant revision.
The immune system, particularly the chronic inflammatory response to a foreign implant material, plays a critical role in the development of ALTR.34 The term chronic inflammation used in the context of ALTR describes the process of persistent, low-grade, long-term inflammation which can last several years. The pathogenesis of chronic inflammation and ALTR can depend on the host immune reaction to the implant material itself and, to implant wear particles.38,39 Other factors, such as, surgery40,41, infection, the patient’s underlying condition and patient-related risk factors can further contribute to and/or aggravate the development of ALTR.42
Orthopedic wear particles trigger an inflammatory response in the periprosthetic environment
Despite the biocompatibility of the materials used and the improvements in implant design, material, and surgical techniques, factors such as mechanical forces, chemical reactions, material degradation, and biological interactions in the joint space can generate micro- and/or nano-scale wear debris originating from the implant bearing surface and junctions, which can trigger an inflammatory response.
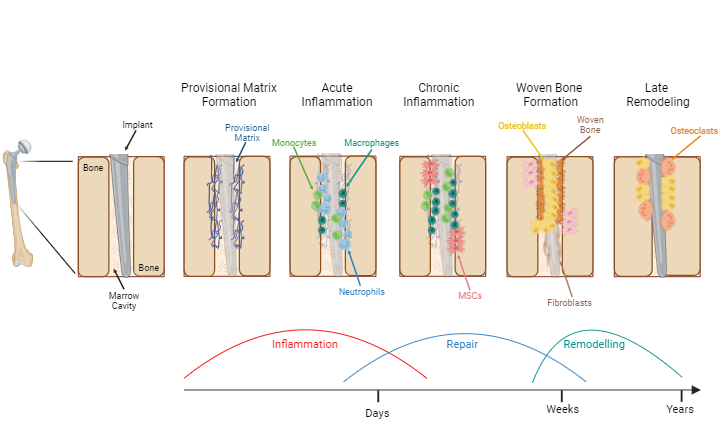
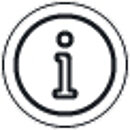
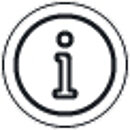
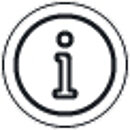
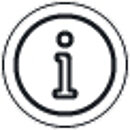
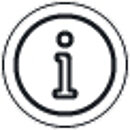
Following TJA, a provisional matrix (blue scaffold) is formed in the peri-implant space.
Macrophages (dark green cells) are activated and release inflammatory cytokines into peri-implant space. Neutrophils (blue cells) and monocytes (light green cells) are attracted and migrate into peri-implant space. Damaged tissue particles are removed.
Monocytes infiltrate into peri-implant space and differentiate into macrophages. Mesenchymal stromal cells (MSCs, pink cells) are recruited.
MSCs differentiate into osteoblasts (yellow cells), forming immature primary woven bone. Fibroblasts (brown cells) adhere on implant surface, forming a fibrous membrane.
Bone remodeling through osteoblasts and osteoclasts (orange cells), eventually transforming the woven bone into lamellar bone.
Following TJA, a provisional matrix (blue scaffold) is formed in the peri-implant space.
Macrophages (dark green cells) are activated and release inflammatory cytokines into peri-implant space. Neutrophils (blue cells) and monocytes (light green cells) are attracted and migrate into peri-implant space. Damaged tissue particles are removed.
Monocytes infiltrate into peri-implant space and differentiate into macrophages. Mesenchymal stromal cells (MSCs, pink cells) are recruited.
MSCs differentiate into osteoblasts (yellow cells), forming immature primary woven bone. Fibroblasts (brown cells) adhere on implant surface, forming a fibrous membrane.
Bone remodeling through osteoblasts and osteoclasts (orange cells), eventually transforming the woven bone into lamellar bone.
Fig. 5:Periprosthetic bone reaction after total or partial joint arthroplasty.
After total or partial joint arthroplasty, periprosthetic bone healing occurs between the implant and the bone surface, properly integrating the implant into bone tissue. The process of periprosthetic bone healing is exemplified with a hip implant in the femoral shaft.
Figure created with BioRender.com, 2024.
Macrophages play a crucial role in wear particle-induced periprosthetic tissue inflammation and bone resorption
Macrophages are the key immune cells responsible for the elimination of wear particles.43 Macrophage activation by wear particles is the dominant mechanism of wear particle-induced periprosthetic soft tissue- and bone inflammation.44 The macrophage reactivity is dependent and driven by chemical and physical features of the particles themselves.45 Macrophages recognize wear particles as foreign material by specific receptors on their cell surface. The wear particles are then engulfed by the cells in processes called endocytosis and phagocytosis, removing them from the tissue. Once engulfed by macrophages, the wear particles either degrade or accumulate in the cell cytoplasm. The activation of the macrophages results in the release of pro-inflammatory mediators, which recruit further immune cells to the site of wear particle accumulation, triggering a local tissue inflammation. Moreover, the release of pro-inflammatory mediators and other factors from macrophages activate bone-resorbing cells (osteoclasts), inducing osteolysis.46,47 Osteolysis is the process of progressive destruction of periprosthetic bone tissue.48 Histopathologic observations from clinical samples and experimental studies indicate that particle-laden macrophages might also be able to directly induce osteolysis by migrating into the bone microenvironment and interacting with osteoclasts.49-51 The macrophages also interact with stroma, endothelial cells of the capillary vessels, and other cell types associated with inflammation.45,52 Depending on particle size, material, and tissue concentration, this reaction can lead to constant activation of macrophages, release of pro-inflammatory mediators, and cell recruitment, resulting in a prolonged and chronic periprosthetic tissue inflammation, periprosthetic osteolysis, and bone resorption.53 Wear particle-induced chronic inflammation and osteolysis are associated with long-term implant failure and aseptic loosening, particularly at later stages after arthroplasty, and can result in revision surgery.45,53,54 Figure 6 depicts a macrophage reaction to wear debris.
In cases of hypersensitivity, the adaptive immune system is activated primarily in response to metal ions, causing an inflammatory response.55-57
Tissue-resident macrophages (green cells) in periprosthetic soft tissue recognize wear debris particles by specific receptors on their surface and become activated. Macrophages start to engulf and digest wear particles by phagocytosis and release inflammatory cytokines (green dots) into the periprosthetic tissue.
Inflammatory cytokines attract other immune cells to the site of wear particle accumulation. Neutrophils (light blue cells) and monocytes (dak red cells) migrate into tissue. Monocytes differentiate into macrophages. Macrophages and neutrophils engulf and digest wear particles.
Depending on size/shape, material and tissue concentration of wear particles, the acute inflammatory response does not resolve and progresses into a chronic tissue inflammation. Monocytes constantly migrate into tissue, differentiating into macrophages. Activated, particle-laden macrophages persistently release pro-inflammatory cytokines into periprosthetic tissue, maintaining chronic tissue inflammation. Furthermore, the released cytokines as well as particle-laden macrophages themselves activate osteoclasts (orange cells), which then start to resorb the periprosthetic bone, resulting in periprosthetic osteolysis.
Tissue-resident macrophages (green cells) in periprosthetic soft tissue recognize wear debris particles by specific receptors on their surface and become activated. Macrophages start to engulf and digest wear particles by phagocytosis and release inflammatory cytokines (green dots) into the periprosthetic tissue.
Inflammatory cytokines attract other immune cells to the site of wear particle accumulation. Neutrophils (light blue cells) and monocytes (dak red cells) migrate into tissue. Monocytes differentiate into macrophages. Macrophages and neutrophils engulf and digest wear particles.
Depending on size/shape, material and tissue concentration of wear particles, the acute inflammatory response does not resolve and progresses into a chronic tissue inflammation. Monocytes constantly migrate into tissue, differentiating into macrophages. Activated, particle-laden macrophages persistently release pro-inflammatory cytokines into periprosthetic tissue, maintaining chronic tissue inflammation. Furthermore, the released cytokines as well as particle-laden macrophages themselves activate osteoclasts (orange cells), which then start to resorb the periprosthetic bone, resulting in periprosthetic osteolysis.
Wear particle-induced formation of Foreign Body Giant Cells (FBGCs)
As mentioned, the formation of so-called foreign body giant cells (FBGCs) is part of the foreign body response (FBR) to an implant during wound healing in subcutaneous tissue but is extremely unusual during periprosthetic wound healing in the joint after TJA. However, FBGCs can form in later stages of wear particle-induced periprosthetic inflammation. They are the consequence of macrophage–macrophage fusion, resulting in a giant cell with multiple cell nuclei. Usually, small wear particles are efficiently degraded and eliminated by macrophages. FBGCs form either when phagocytosis is an insufficient primary mechanism of material degradation or when wear particles or when agglomerates/aggregates are in a size range between 10-100 µm.20,58,59
The term ‘frustrated phagocytosis’ describes the formation of FBGCs in presence of orthopedic cement or very large particles, which impair FBGC function and thus inhibit phagocytosis of these large particles. Importantly, FBGCs have also been found in granulomatous diseases in absence of any particulate debris. Figure 7 shows the formation of FBGCs.
A small debris particle (purple particle) is recognized by a macrophage (green cell). The macrophage starts to engulf and phagocytose the particle, resulting in the digestion of the particle. These particle-laden macrophages are further transported in periprosthetic bone tissue and marrow and in periprosthetic soft tissue.
A large debris particle is recognized by a macrophage but cannot be digested because of its size. This leads to the accumulation and fusion of multiple macrophages around the large particle. The fusion of multiple macrophages results in the formation of a multinucleated giant cell, and in some cases in frustrated phagocytosis.
A small debris particle (purple particle) is recognized by a macrophage (green cell). The macrophage starts to engulf and phagocytose the particle, resulting in the digestion of the particle. These particle-laden macrophages are further transported in periprosthetic bone tissue and marrow and in periprosthetic soft tissue.
A large debris particle is recognized by a macrophage but cannot be digested because of its size. This leads to the accumulation and fusion of multiple macrophages around the large particle. The fusion of multiple macrophages results in the formation of a multinucleated giant cell, and in some cases in frustrated phagocytosis.
The immunological profile of different orthopedic wear particles
Wear particles from orthopedic implants have been shown to cause an inflammatory response in the periprosthetic tissue (fig. 8).60 Determination of implant wear (particulate and non-particulate state) in soft tissue and in bone or bone marrow is important to link the observed adverse tissue reactions to the implant. Local particle presence has mostly been studied in the peri-implant membrane and synovial fluid, but not comprehensively in the adjacent bone and bone marrow due to limited availability of clinical samples.
The shape and type of released wear particles influence the inflammatory response,45,61 and tissue alterations and morphology differ macroscopically and microscopically.62 The type of implant material is the driving factor for the development of chronic inflammation. There are differences in the immuno-compatibility of orthopedic materials, which appear to differently shape the peri-implant microenvironment.49 The following section describes different orthopedic implant materials and the immunological profile of their wear particles:
Ultra-high-molecular-weight polyethylene (UHMWPE) particles activate macrophages by different inflammatory signaling pathways63 and initiate the release of pro-inflammatory mediators, causing osteoclastogenesis and bone resorption.64 UHMWPE generates relatively large amounts of volumetric wear when interfacing with the metallic head of hip implants. Therefore, carbon crosslinking methods such as gamma irradiation, chemical induction, and addition of antioxidant agents have been implemented to increase material wear resistance65 in an attempt to minimize the pro-inflammatory response and incidence of complications.66
The generation of metallic debris is still a matter of great concern regarding periprosthetic osteolysis. Cobalt, nickel, and titanium implant particles were shown to activate macrophages via different inflammatory signaling pathways,70-74 resulting in periprosthetic chronic inflammation and osteolysis. As mentioned above, metal ions were also shown to cause a delayed type of hypersensitivity by activating cells of the adaptive immune system (T-cells), which may also contribute to aseptic loosening.55,57,75-77 However, only experimental studies have been performed so far. Whether the adaptive immune system additionally contributes to metal particles/ions-induced aseptic loosening is still a matter of intense debate, and more studies are needed for clarification.
Ceramic-on-ceramic implants have been proposed as the best option for young and active patients.78,79 These implants display minimal wear-debris generation, with limited incidence of osteolysis and long-term survival rates.78,79 Alumina has been shown to have a low cellular immunotoxicity. Alumina particles were further demonstrated to have only limited capacity to stimulate the release of pro-inflammatory mediators from human macrophages80, and high concentrations of alumina ceramic particles induced only a weak up-regulation of mediators for osteoclastogenesis.81 Also, pathogenic reactions to ceramic wear particles are considered as unlikely.82 Moreover, alumina is considered hypoallergenic material, providing a valuable alternative for patients with metal hypersensitivity.83,84
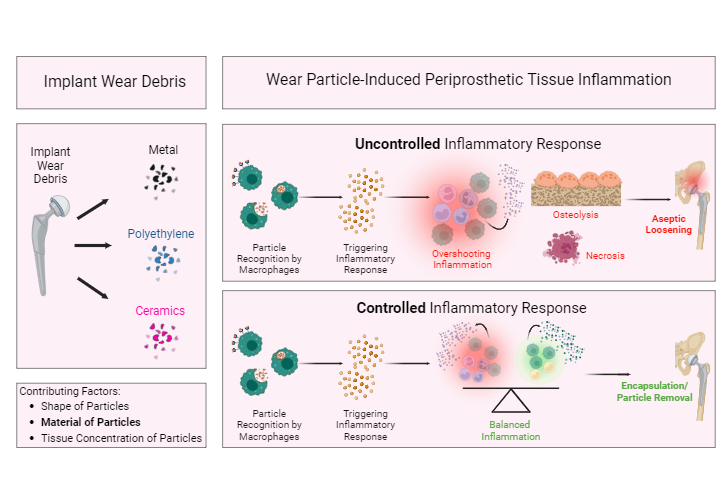
Fig. 8: Implant wear debris and wear particle-induced periprosthetic tissue inflammation.
Wear debris is generated from the bearing surface of an implant. Based on the bearing surface, the wear debris can be of different material, such as metal, polyethylene, or ceramics. The contributing factors for the development of wear particle-induced periprosthetic tissue inflammation are the particles’ shape and size, tissue concentration, and material. The material is the driving force for tissue inflammation. Wear particles in periprosthetic tissue are considered foreign bodies and thus trigger an inflammatory response. Wear debris particles (orange particles) in periprosthetic tissue are recognized by macrophages (green cells) via specific surface receptors. Macrophages are activated and phagocytose these particles. Activated macrophages release inflammatory cytokines (orange dots), triggering an inflammatory tissue response. Depending on particle shape/size, tissue concentration, and material, this can result in an overshooting and chronic inflammatory response, causing tissue necrosis and osteolysis. An uncontrolled inflammatory response is associated with aseptic loosening of the implant. A controlled wear debris-induced inflammatory response is characterized by a well-balanced pro- and anti-inflammatory response, which eventually results in encapsulation of the implant and in particle removal.
Figure created with BioRender.com, 2024.
Conclusion
In conclusion, inflammation is an essential, multifactorial but complex whole-body response that can have harmful effects under certain conditions. Inflammation plays a crucial role in wound healing after TJA but is also associated with the development of adverse local tissue reactions (ALTRs), which can lead to implant revision. While early-stage ALTRs are related to surgical techniques, implant material and design, and patient-related risk factors and predispositions, late-stage ALTRs are associated with chronic inflammation caused by wear debris from the implant. The best solution to avoid multiple revisions due to ALTR is prevention by avoiding the use of biomaterials that promote ALTR. This can be achieved by choosing biomaterials with low toxicity, high biocompatibility, hypoallergenic properties, and low pro-inflammatory potential, such as (for example) ceramics.
Summary
Inflammation is a natural, whole-body response triggered by the immune system. The immune system performs essential functions such as combating pathogens, eliminating foreign bodies, initiating wound and fracture healing, initiating tissue repair and reconstitution, and re-establishing tissue homeostasis after injury. The inflammatory response is a complex but tightly regulated process involving many cells and signaling pathways. The acute inflammatory response is a strictly regulated but temporarily limited process; but it can turn into long-term, low-grade chronic inflammation, which is an undesirable and harmful process.
One must take caution when talking about chronic inflammation. The term is often used for different inflammatory processes, which is why it is very important to specify the respective process. As explained, chronic inflammation during wound healing after TJA differs from chronic inflammation to implanted materials as part of FBR.
TJA is the ultimate solution to replace and preserve the form and function of major joints. The inflammatory response is an indispensable process of wound healing after TJA and the proper reconstruction of tissue at the implant site, which usually resolves within a few weeks. However, patient-related risk factors, pathologic predispositions, surgical techniques, hypersensitivity, orthopedic material, and the presence of implant wear particles can trigger an adverse local tissue reaction (ALTR) associated with low-grade chronic inflammation in periprosthetic tissue, osteolysis and bone resorption. This can result in aseptic loosening, periprosthetic implant failure, and revision surgery.
Wear particles from various orthopedic implant materials have been shown to trigger a local inflammatory reaction, with ceramic particles being the least reactive and therefore only triggering a mild and controlled pro-inflammatory reaction.
The best solution to avoid ALTR-related multiple revisions is prevention by avoiding the use of biomaterials that promote ALTR. Some orthopedic implant materials have a higher likelihood of triggering ALTR due to their physicochemical properties or may lead to potential exacerbation in combination with other material and patient-related risk factors.
- Bennett JM, Reeves G, Billman GE, Sturmberg JP. Inflammation–Nature's way to efficiently respond to all types of challenges: implications for understanding and managing “the Epidemic” of chronic diseases. Front Med (Lausanne). 2018;5:316. doi:10.3389/fmed.2018.00316.C
- Chen L, Deng H, Cui H, et al. Inflammatory responses and inflammation-associated diseases in organs. Oncotarget. 2017;9(6):7204-7218. doi:10.18632/oncotarget.23208.
- Medzhitov R. Inflammation 2010: new adventures of an old flame. Cell. 2010;140(6):771-776. doi:10.1016/j.cell.2010.03.006.
- Medzhitov R. Origin and physiological roles of inflammation. Nature. 2008;454(7203):428-435. doi:10.1038/nature07201.
- Hannoodee S, Nasuruddin DN. Acute Inflammatory Response. StatPearls. Treasure Island (FL): StatPearls Publishing LLC; 2024.
- Pahwa R, Goyal A, Jialal I. Chronic Inflammation. StatPearls. Treasure Island (FL): StatPearls Publishing LLC.; 2024.
- Cavaillon JM. Once upon a time, inflammation. J Venom Anim Toxins Incl Trop Dis. 2021;27:e20200147. doi:10.1590/1678-9199-JVATITD-2020-0147.
- Rather LJ. Disturbance of function (functio laesa): the legendary fifth cardinal sign of inflammation, added by Galen to the four cardinal signs of Celsus. Bull N Y Acad Med. 1971;47(3):303-322.
- Punchard NA, Whelan CJ, Adcock I. The journal of inflammation. J Inflamm (Lond). 2004;1(1):1. doi:10.1186/1476-9255-1-1.
- Gibon E, Takakubo Y, Zwingenberger S, Gallo J, Takagi M, Goodman SB. Friend or foe? Inflammation and the foreign body response to orthopedic biomaterials. J Biomed Mater Res A. 2023;112(8):1172-1187. doi:10.1002/jbm.a.37599.
- Medzhitov R, Janeway C. Innate immunity. N Engl J Med. 2000;343(5):338-344. doi:10.1056/NEJM200008033430506.
- Signore A. About inflammation and infection. EJNMMI Res. 2013;3(1):8. doi:10.1186/2191-219X-3-8.
- Soliman AM, Barreda DR. Acute inflammation in tissue healing. Int J Mol Sci. 2023;24(1):641. doi:10.3390/ijms24010641.
- Serhan CN, Savill J. Resolution of inflammation: the beginning programs the end. Nat Immunol. 2005;6(12):1191-1197. doi:10.1038/ni1276.
- Kawai T, Akira S. The role of pattern-recognition receptors in innate immunity: update on Toll-like receptors. Nat Immunol. 2010;11(5):373-384. doi:10.1038/ni.1863.
- Uribe-Querol E, Rosales C. Phagocytosis: our current understanding of a universal biological process. Front Immunol. 2020;11:1066. doi:10.3389/fimmu.2020.01066.
- Levin R, Grinstein S, Canton J. The life cycle of phagosomes: formation, maturation, and resolution. Immunol Rev. 2016;273(1):156-179. doi:10.1111/imr.12439.
- Wynn TA, Vannella KM. Macrophages in tissue repair, regeneration, and fibrosis. Immunity. 2016;44(3):450-462. doi:10.1016/j.immuni.2016.02.015.
- Londono R, Badylak SF. Chapter 1 - Factors Which Affect the Host Response to Biomaterials. In: Badylak SF, ed. Host Response to Biomaterials. Oxford: Academic Press; 2015:1-12.
- Anderson JM, Rodriguez A, Chang DT. Foreign body reaction to biomaterials. Semin Immunol. 2008;20(2):86-100. doi:10.1016/j.smim.2007.11.004.
- Anderson J, Cramer S. Chapter 2 - Perspectives on the Inflammatory, Healing, and Foreign Body Responses to Biomaterials and Medical Devices. In: Badylak SF, ed. Host Response to Biomaterials. Oxford: Academic Press; 2015:13-36.
- Guo S, DiPietro LA. Factors affecting wound healing. J Dent Res. 2010;89(3):219-229. doi:10.1177/0022034509359125.
- Anderson JM, Jiang S. Implications of the Acute and Chronic Inflammatory Response and the Foreign Body Reaction to the Immune Response of Implanted Biomaterials. In: Corradetti B, ed. The Immune Response to Implanted Materials and Devices: The Impact of the Immune System on the Success of an Implant. Cham: Springer International Publishing; 2017:15-36.
- Klopfleisch R, Jung F. The pathology of the foreign body reaction against biomaterials. J Biomed Mater Res A. 2017;105(3):927-940. doi:10.1002/jbm.a.35958.
- Moore LB, Kyriakides TR. Molecular Characterization of Macrophage-Biomaterial Interactions. In: Lambris JD, Ekdahl KN, Ricklin D, Nilsson B, eds. Immune Responses to Biosurfaces, Advances in Experimental Medicine and Biology. Springer; 2015:109-122.
- Kuzyk PR, Schemitsch EH. The basic science of peri-implant bone healing. Indian J Orthop. 2011;45(2):108-115. doi:10.4103/0019-5413.77129.
- Liu Y, Rath B, Tingart M, Eschweiler J. Role of implants surface modification in osseointegration: a systematic review. J Biomed Mater Res A. 2020;108(3):470-484. doi:10.1002/jbm.a.36829.
- Choi JY, Sim JH, Yeo IL. Characteristics of contact and distance osteogenesis around modified implant surfaces in rabbit tibiae. J Periodontal Implant Sci. 2017;47(3):182-192. doi:10.5051/jpis.2017.47.3.182.
- Morozov P, Sana M, McGrory BJ, Farraher SW, Abrahams TG. Comparison of pre-revision magnetic resonance imaging and operative findings in mechanically assisted crevice corrosion in symptomatic metal on polyethylene total hip replacements. J Arthroplasty. 2017;32(8):2535-2545. doi:10.1016/j.arth.2017.03.021.
- Nawabi DH, Gold S, Lyman S, Fields K, Padgett DE, Potter HG. MRI predicts ALVAL and tissue damage in metal-on-metal hip arthroplasty. Clin Orthop Relat Res. 2014;472(2):471-481. doi:10.1007/s11999-013-2788-y.
- Ricciardi BF, Nocon AA, Jerabek SA, et al. Histopathological characterization of corrosion product associated adverse local tissue reaction in hip implants: a study of 285 cases. BMC Clin Pathol. 2016;16(3):eCollection 2016. doi:10.1186/s12907-016-0025-9.
- Williams DH, Greidanus NV, Masri BA, Duncan CP, Garbuz DS. Prevalence of pseudotumor in asymptomatic patients after metal-on-metal hip arthroplasty. J Bone Joint Surg Am. 2011;93(23):2164-2171. doi:10.2106/jbjs.J.01884.
- Almousa SA, Greidanus NV, Masri BA, Duncan CP, Garbuz DS. The natural history of inflammatory pseudotumors in asymptomatic patients after metal-on-metal hip arthroplasty. Clin Orthop Relat Res. 2013;471(12):3814-3821. doi:10.1007/s11999-013-2944-4.
- Eltit F, Wang Q, Wang R. Mechanisms of adverse local tissue reactions to hip implants. Front Bioeng Biotechnol. 2019;7(176):eCollection 2019. doi:10.3389/fbioe.2019.00176.
- Mahendra G, Pandit H, Kliskey K, Murray D, Gill HS, Athanasou N. Necrotic and inflammatory changes in metal-on-metal resurfacing hip arthroplasties. Acta Orthop. 2009;80(6):653-659. doi:10.3109/17453670903473016.
- Natu S, Sidaginamale RP, Gandhi J, Langton DJ, Nargol AV. Adverse reactions to metal debris: histopathological features of periprosthetic soft tissue reactions seen in association with failed metal on metal hip arthroplasties. J Clin Pathol. 2012;65(5):409-418. doi:10.1136/jclinpath-2011-200398.
- Perino G, Ricciardi BF, Jerabek SA, et al. Implant based differences in adverse local tissue reaction in failed total hip arthroplasties: a morphological and immunohistochemical study. BMC Clin Pathol. 2014;14(1):39. doi:10.1186/1472-6890-14-39.
- Billi F, Benya P, Kavanaugh A, Adams J, McKellop H, Ebramzadeh E. The John Charnley Award: an accurate and extremely sensitive method to separate, display, and characterize wear debris: part 2: metal and ceramic particles. Clin Orthop Relat Res. 2012;470(2):339-350. doi:10.1007/s11999-011-2058-9.
- Sonntag R, Reinders J, Kretzer JP. Bio-Tribological Demands. In: Sonntag R, Kretzer JP, eds. Materials for Total Joint Arthroplasty. World Scientific; 2016:1-13.
- Thapa P, Euasobhon P. Chronic postsurgical pain: current evidence for prevention and management. Korean J Pain. 2018;31(3):155-173. doi:10.3344/kjp.2018.31.3.155.
- Amstutz HC, Le Duff MJ, Johnson AJ. Socket position determines hip resurfacing 10-year survivorship. Clin Orthop Relat Res. 2012;470(11):3127-3133. doi:10.1007/s11999-012-2347-y.
- Vendittoli PA, Riviere C, Hirschmann MT, Bini S. Why personalized surgery is the future of hip and knee arthroplasty: a statement from the Personalized Arthroplasty Society. EFORT Open Rev. 2023;8(12):874-882. doi:10.1530/eor-22-0096.
- Nich C, Takakubo Y, Pajarinen J, et al. Macrophages—Key cells in the response to wear debris from joint replacements. J Biomed Mater Res A. 2013;101(10):3033-3045. doi:10.1002/jbm.a.34599.
- Athanasou NA. The pathobiology and pathology of aseptic implant failure. Bone Joint Res. 2016;5(5):162-168. doi:10.1302/2046-3758.55.bjr-2016-0086.
- Couto M, Vasconcelos DP, Sousa DM, et al. The mechanisms underlying the biological response to wear debris in periprosthetic inflammation. Front Mater Sci. 2020;7:1-13. doi:10.3389/fmats.2020.00274.
- Landgraeber S, Jäger M, Jacobs JJ, Hallab NJ. The pathology of orthopedic implant failure is mediated by innate immune system cytokines. Mediators Inflamm. 2014;2014:185150. doi:10.1155/2014/185150.
- Pan B, Zhang Z, Wu X, et al. Macrophage-derived exosomes modulate wear particle-induced osteolysis via miR-3470b targeting TAB3/NF-κB signaling. Bioact Mater. 2023;26:181-193. doi:10.1016/j.bioactmat.2023.02.028.
- Saleh KJ, Schwarz EM. Osteolysis: medical and surgical approaches. Clin Orthop Relat Res. 2004;427:138-147.
- Panez-Toro I, Heymann D, Gouin F, Amiaud J, Heymann M-F, Córdova LA. Roles of inflammatory cell infiltrate in periprosthetic osteolysis. Front Immunol. 2023;14:1310262. doi:10.3389/fimmu.2023.1310262.
- Yin Z, Gong G, Liu X, Yin J. Mechanism of regulating macrophages/osteoclasts in attenuating wear particle-induced aseptic osteolysis. Front Immunol. 2023;14:1274679. doi:10.3389/fimmu.2023.1274679.
- Nich C, Goodman SB. Role of macrophages in the biological reaction to wear debris from joint replacements. J Long Term Eff Med Implants. 2014;24(4):259-265. doi:10.1615/jlongtermeffmedimplants.2014010562.
- Gibon E, Cordova LA, Lu L, et al. The biological response to orthopedic implants for joint replacement. II: Polyethylene, ceramics, PMMA, and the foreign body reaction. J Biomed Mater Res B Appl Biomater. 2017;105(6):1685-1691. doi:10.1002/jbm.b.33676.
- Goodman SB, Gallo J. Periprosthetic osteolysis: mechanisms, prevention and treatment. J Clin Med. 2019;8(12):2091. doi:10.3390/jcm8122091.
- Connors JP, Stelzer JW, Garvin PM, Wellington IJ, Solovyova O. The role of the innate immune system in wear debris-induced inflammatory peri-implant osteolysis in total joint arthroplasty. Bioengineering (Basel). 2022;9(12):764. doi:10.3390/bioengineering9120764.
- Goodman SB. Wear particles, periprosthetic osteolysis and the immune system. Biomaterials. 2007;28(34):5044-5048. doi:10.1016/j.biomaterials.2007.06.035.
- Pajarinen J, Jamsen E, Konttinen YT, Goodman SB. Innate immune reactions in septic and aseptic osteolysis around hip implants. J Long Term Eff Med Implants. 2014;24(4):283-296. doi:10.1615/jlongtermeffmedimplants.2014010564.
- Schalock PC, Crawford G, Nedorost S, et al. Patch testing for evaluation of hypersensitivity to implanted metal devices: a perspective from the American Contact Dermatitis Society. Dermatitis. 2016;27(5):241-247. doi:10.1097/der.0000000000000210.
- Eslami-Kaliji F, Hedayat Nia N, Lakey JRT, Smink AM, Mohammadi M. Mechanisms of foreign body giant cell formation in response to implantable biomaterials. Polymers (Basel). 2023;15(5):1313. doi:10.3390/polym15051313.
- Xia Z, Triffitt JT. A review on macrophage responses to biomaterials. Biomedical materials (Bristol, England). 2006;1(1):R1-9. doi:10.1088/1748-6041/1/1/r01.
- Gibon E, Goodman SB. The biologic response to bearing materials. Orthopaedic knowledge online. 2016;14(6)
- Goodman SB, Gallo J, Gibon E, Takagi M. Diagnosis and management of implant debris-associated inflammation. Expert Rev Med Devices. 2020;17(1):41-56. doi:10.1080/17434440.2020.1702024.
- Perino G, Sunitsch S, Huber M, et al. Diagnostic guidelines for the histological particle algorithm in the periprosthetic neo-synovial tissue. BMC Clin Pathol. 2018;18:7. doi:10.1186/s12907-018-0074-3.
- Maitra R, Clement CC, Scharf B, et al. Endosomal damage and TLR2 mediated inflammasome activation by alkane particles in the generation of aseptic osteolysis. Mol Immunol. 2009;47(2-3):175-184. doi:10.1016/j.molimm.2009.09.023.
- Terkawi MA, Hamasaki M, Takahashi D, et al. Transcriptional profile of human macrophages stimulated by ultra-high molecular weight polyethylene particulate debris of orthopedic implants uncovers a common gene expression signature of rheumatoid arthritis. Acta Biomater. 2018;65:417-425. doi:10.1016/j.actbio.2017.11.001.
- Bracco P, Bellare A, Bistolfi A, Affatato S. Ultra-high molecular weight polyethylene: influence of the chemical, physical and mechanical properties on the wear behavior. A review. Materials. 2017;10(7):791. doi:10.3390/ma10070791.
- Broomfield JAJ, Malak TT, Thomas GER, Palmer AJR, Taylor A, Glyn-Jones S. The relationship between polyethylene wear and peri-prosthetic osteolysis In total hip arthroplasty at 12 years in a randomized controlled trial cohort. J Arthroplasty. 2017;32(4):1186-1191. doi:10.1016/j.arth.2016.10.037.
- Pearl JI, Ma T, Irani AR, et al. Role of the Toll-like receptor pathway in the recognition of orthopedic implant wear-debris particles. Biomaterials. 2011;32(24):5535-5542. doi:10.1016/j.biomaterials.2011.04.046.
- Antonios JK, Yao Z, Li C, Rao AJ, Goodman SB. Macrophage polarization in response to wear particles in vitro. Cell Mol Immunol. 2013;10(6):471-482. doi:10.1038/cmi.2013.39.
- Burton L, Paget D, Binder NB, et al. Orthopedic wear debris mediated inflammatory osteolysis is mediated in part by NALP3 inflammasome activation. J Orthop Res. 2013;31(1):73-80. doi:10.1002/jor.22190.
- Caicedo MS, Samelko L, McAllister K, Jacobs JJ, Hallab NJ. Increasing both CoCrMo-alloy particle size and surface irregularity induces increased macrophage inflammasome activation in vitro potentially through lysosomal destabilization mechanisms. J Orthop Res. 2013;31(10):1633-1642. doi:10.1002/jor.22411.
- Samelko L, Landgraeber S, McAllister K, Jacobs J, Hallab NJ. Cobalt alloy implant debris induces inflammation and bone loss primarily through danger signaling, not TLR4 activation: implications for DAMP-ening implant related inflammation. PLoS One. 2016;11(7):e0160141. doi:10.1371/journal.pone.0160141.
- Eger M, Hiram-Bab S, Liron T, et al. Mechanism and prevention of titanium particle-induced inflammation and osteolysis. Front Immunol. 2018;9:2963. doi:10.3389/fimmu.2018.02963.
- Baron L, Gombault A, Fanny M, et al. The NLRP3 inflammasome is activated by nanoparticles through ATP, ADP and adenosine. Cell death & disease. 2015;6(2):e1629. doi:10.1038/cddis.2014.576.
- Jämsen E, Pajarinen J, Kouri VP, et al. Tumor necrosis factor primes and metal particles activate the NLRP3 inflammasome in human primary macrophages. Acta Biomater. 2020;108:347-357. doi:10.1016/j.actbio.2020.03.017.
- Pajarinen J, Lin TH, Sato T, Yao Z, Goodman SB. Interaction of materials and biology in total joint replacement – successes, challenges and future directions. J Mater Chem B. 2014;2(41):7094-7108. doi:10.1039/C4TB01005A.
- Lu L, Vollmer J, Moulon C, Weltzien HU, Marrack P, Kappler J. Components of the ligand for a Ni++ reactive human T cell clone. J Exp Med. 2003;197(5):567-574. doi:10.1084/jem.20021762.
- Clayton GM, Wang Y, Crawford F, et al. Structural basis of chronic beryllium disease: linking allergic hypersensitivity and autoimmunity. Cell. 2014;158(1):132-142. doi:10.1016/j.cell.2014.04.048.
- Hamadouche M, Boutin P, Daussange J, Bolander ME, Sedel L. Alumina-on-alumina total hip arthroplasty: a minimum 18.5-year follow-up study. J Bone Joint Surg Am. 2002;84-A(1):69-77.
- Hannouche D, Devriese F, Delambre J, et al. Ceramic-on-ceramic THA implants in patients younger than 20 years. Clin Orthop Relat Res. 2016;474(2):520-527. doi:10.1007/s11999-015-4546-9.
- Kaufman AM, Alabre CI, Rubash HE, Shanbhag AS. Human macrophage response to UHMWPE, TiAlV, CoCr, and alumina particles: analysis of multiple cytokines using protein arrays. J Biomed Mater Res A. 2008;84(2):464-474. doi:10.1002/jbm.a.31467.
- Bylski D, Wedemeyer C, Xu J, Sterner T, Loer F, von KM. Alumina ceramic particles, in comparison with titanium particles, hardly affect the expression of RANK-, TNF-alpha-, and OPG-mRNA in the THP-1 human monocytic cell line. J Biomed Mater Res A. 2009;89(3):707-716. doi:10.1002/jbm.a.31956.
- Krenn V, Thomas P, Thomsen M, et al. Histopathologische Partikelidentifikation (Partikelalgorithmus nach Krenn). CeraNews2013. p. 12-17.
- Thomas P, Stea S. Metal Implant Allergy and Immuno-Allergological Compatibility Aspects of Ceramic Materials. Clinical Management of Joint Arthroplasty. Heidelberg: Springer-Verlag; 2015.
- van der Merwe JM. Metal hypersensitivity in joint arthroplasty. J Am Acad Orthop Surg Glob Res Rev. 2021;5(3):e20.00200. doi:10.5435/JAAOSGlobal-D-20-00200.